Well depth extended in geothermal project using controlled pressure drilling
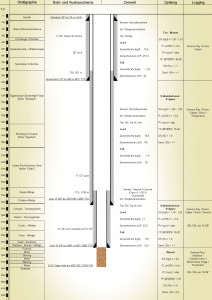
Underbalanced runs prevent mud losses and reservoir damage, allow well to hit main fault
By Essam Sammat, Stephen O’Shea, Gareth Innes, Weatherford UK; Julio Kemenyfy, Darko Piscevic, GEOenergie Bayern
Wells in the Kirchweidach geothermal project in Bavaria, Germany, and other offset wells in the area have faced problems such as severe mud losses and differential sticking in the reservoir formation. However, control pressure drilling (CPD) was successfully applied to address those challenges.
The project’s objective was to erect a power plant that would produce 6 to 8 MW of electricity and supply the local town and industries with district heating using thermal energy. Two wells were planned targeting natural fractures in the Malm formation (Jurassic carbonate), including one producer and one injector. This would allow more than 90% of the produced water to be returned to the reservoir, in time making the project sustainable.
The first geothermal well was drilled using CPD equipment in the reservoir section from the beginning. The underbalanced borehole pressure was achieved by pumping various rates of nitrogen and fresh water with polymers, which can significantly reduce nonproductive time and formation damage. For the second well, CPD equipment was used only after mud losses appeared.
The Malm formation is an underpressured aquifer that is often karstified, which at times resulted in severe or total fluid losses in the wells crossing it. In Kirchweidach, the top of Malm is at around 3,450 meters TVD, 400-meters thick and 130°C. As fluid losses during drilling are an indicator of success for the project, a procedure was implemented to allow drilling under these conditions to reach all targets while keeping the reservoir as clean as possible.
The Kirchweidach wells have the longest open-hole sections for geothermal wells, with GT 1 at 1,276 meters, GT 2 at 1,241 meters and GT 2a at 1,378 meters. They are also the only horizontal wells drilled in this formation. At the top of Malm, the separation between GT 1 and GT 2a is 1,600 meters. Figure 1 shows the typical well design, and Figure 2 shows the structural placement of the wells.
Controlled Pressure Drilling
Controlled pressure drilling uses a closed and pressured wellbore instead of drilling with the hole “open” to the atmosphere. A rotating control device (RCD) closes the well at surface, allowing for more precise control of the pressure profile. The RCD directs the flow of cuttings brought up by the aerated/nitrified fluid from the rig to the geothermal separator.
To do this, the flowline from wellhead to separator connects to a drilling spool below the RCD. This facility also provides the option of flowing cold water over the top of the well to stay within RCD rubber element temperature specifications if necessary.
The rubber seal unit rotates with and seals around the drill pipe and tool joint when drilling, making connections or tripping in or out of the hole.
The three main types of CPD methods are air drilling (AD), managed pressure drilling (MPD) and underbalanced drilling (UBD). AD is geared toward increasing the rate of penetration (ROP), MPD reduces rig non-performance time, and UBD minimizes reservoir damage and increases productivity.
Well GT 1 was drilled using two of these CPD methods as it varied temporarily from at-balance to underbalance conditions using nitrified fresh water, with the intention to avoid continuous influx to surface. Accordingly, the drilling method could justifiably be termed CPD, MPD or UBD. For this article, the four runs undertaken will be referred to as UBD Runs 1, 2, 3 and 4 even though the well was not strictly continuously in underbalance conditions.
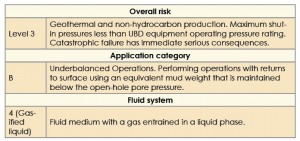
Planning
Well Classification
IADC’s well classification system for underbalanced operations and managed pressure drilling (MPD) describes a well using a three-digit code based on overall risk, application category and fluid system.
Based on this system, GT 1 was planned as 3-B-4:
• Overall risk was Level 3: geothermal and non-hydrocarbon production. Maximum shut-in pressures less than UBD equipment operating pressure rating. Catastrophic failure has immediate serious consequences.
• Application category is Category B: underbalanced operations. Performing operations with returns to surface using an equivalent mud weight that is maintained below the open-hole pore pressure.
• Fluid system is 4 (gasified liquid): fluid medium with a gas entrained in a liquid phase.

Objectives
The objective of GT 1 was to drill the 9 ½-in. open hole to TD using CPD methods with the following criteria:
• Drill the open hole with a two-phase water and nitrified fluid to maintain CPD conditions in the open hole, avoiding reservoir damage;
• Avoid drilling problems such as mud losses, differential sticking and potential kicks by proper fluid control and measurement; and
• Allow potentially faster ROP and lower total drilling days. This was a secondary objective compared with the primary objective of avoiding losses.
By successfully using CPD methods, the following results may also be possible:
• Drill to TD with full returns, allowing collection of geological information;
• Reservoir/production evaluation and characterization while drilling; and
• Gather data for drilling performance optimization and future well planning.
Modeling
Pre-job and rig-site modeling of multiphase flow was done using an advanced simulator to determine the required underbalanced drilling conditions. These include the following input parameters:
1. Gas-to-liquid ratios are evaluated and selected to reduce the hydrostatic pressure within the annulus to achieve the desired bottomhole circulating pressure.
2. Minimum liquid flow velocities, which determine the cutting-carrying/hole-cleaning capacities. By experience, the values for minimum hole-cleaning capacities for water-based mud are:
• Well trajectory: minimum required velocity;
• Horizontal: 55 meters/min; and
• Vertical: 45 meters/min;
3. The mud motor equivalent liquid volume (ELV) is taken into consideration. This value cannot be exceeded.
4. The gas volume fraction (GVF) in the drill pipe can affect downhole tool performance.
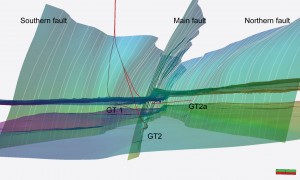
Modeling was performed using data provided by GEOenergie Bayern and known physical constants. To function, Neotec requires a number of input values, including the specific gravity of the intended drilling fluid, composition of the injected gas, borehole trajectory and annular design. Additionally, drill string design, including tubular profiles and operating limits (pressure drop and max motor ELV), are of interest as points of increased annular friction or pressure drop, which can affect downhole fluid velocity.
Estimations are substituted. Initial indications were that target formation pressure was 351 bar (5,089 psi) and formation temperature was 145°C (293°F). Reservoir pressure was thought to be 383 bar (5,555 psi) at TD. Offset wells reported partial to total loss scenarios when drilling with 1.02 to 1.05 sg (8.4 to 8.7 ppg).
A primary requirement of the CPD operation was to reduce annular friction pressure, which is responsible for increases in the bottomhole pressure and the potential for fluid loss. A solution was to establish a high nitrogen injection rate with a moderate fluid injection rate. This also increases the fluid velocity, which aids hole cleaning. When working in a very narrow pressure window, the case where the well is not producing at the casing shoe is considered the worst-case scenario.
Fluid injection rates were designed to lie within the capabilities of the equipment available while not exceeding reservoir pressure draw-down of 10%. Initial modeling was conducted with rates varying from nitrogen at 18 cu meters/min to 28 cu meters/min and fluid injection at 2,000 lpm to 2,600 lpm.
An operation envelope was created that identified an optimal injection rate of 22.6 cu meters/min of nitrogen and 2,400 lpm of drilling fluid with a density of 1.02 sg. This produced a reservoir draw-down of 4 bar (58 psi) while remaining within operating limits of less than 18% GVF (5%) and motor ELV limits.
However, these injection rates provide hole-cleaning velocities of 41 meters/min in the vertical section. Experience has shown that under these conditions, adequate hole cleaning can be achieved through the scheduled pumping of high-viscosity pills, reciprocating the drill string prior to connections and low ROPs. On the other hand, should the reservoir flow, vertical and horizontal fluid velocity would exceed their minimum thresholds, and hole cleaning would be vastly improved.
While concentric casing and parasitic string injection methods were known to be highly effective nitrogen injection methods, drill pipe injection was chosen as it was shown to be adequate.
Nitrogen
There are two methods for getting the required supply of nitrogen on the rig site.
Cryogenic nitrogen is widely used in drilling operations as it is transported to the well site as a liquid, and the boiling point of liquid nitrogen is -196.1°C (-321°F) at atmospheric pressure. Cryogenic tanks are necessary for transportation and storage on location.
Because the nitrogen is pumped as a liquid and the conversion from liquid volume to gas volume at standard conditions is well characterized, it is straightforward to accurately measure and control the nitrogen delivery rate. This also comes with a guaranteed nitrogen purity of 99%, which vastly reduces corrosion effects on equipment.
Membrane nitrogen involves stripping nitrogen molecules from the local atmosphere. This system has different equipment requirements to the cryogenic method, but once the sourced nitrogen is in the standpipe, it provides the exact same function.
Regardless of the nitrogen source, it eliminates the possibility of downhole fires. Pure cryogenic nitrogen also prevents downhole corrosion due to the purity level. Membrane-generated nitrogen contains some oxygen, and downhole corrosion remains a concern. Awareness of corrosion effects is crucial to safe operations and equipment maintenance. Other factors need to be considered before it is decided to use cryogenic or membrane nitrogen, such as cost, availability, site layout and available space, diesel consumption, and noise control.
Based on the above criteria, the plan was to drill GT 1 using cryogenic nitrogen.
Development
Equipment Selection
The CPD geothermal package was designed to have an efficient and minimal on-site footprint. The Model 9000 RCD was perfectly suited for the well conditions projected with 34 bar (500 psi) operating pressure rating, and they close the annulus to the rig floor. RCDs are not well control equipment, and no CPD equipment was labeled as such.
An adapter and two drilling spools were installed between the top of the blowout preventer and the base of the RCD. One of the drilling spools had outlets to connect to the 8-in. flow line and the injection of cold water across the top of the well. The purpose was to ensure heated fluids did not decrease the expected life span of the rubber sealing element.
Between the wellhead and the geothermal separator, a globe valve was installed to regulate flow from the well to stem intermittent slugging from the annulus that was expected to occur. In the top-hole section of the annulus, nitrogen becomes free to rapidly expand due to a decrease in hydrostatic pressure, resulting in slugging at surface. The globe valve was a simplified and recognized method of manually applying surface back pressure to control the release of this fluid.
An 8-in. flow line and a geothermal separator with adjustable frame to match the shaker tank height complete the return flow system. The geothermal separator is where the nitrified drilling fluid is first exposed to open atmosphere and was designed to effectively allow the separation of nitrogen from the drilling fluid. This equipment employs the principle of centrifugal force for liquid-gas separation as in cyclone equipment. The nitrogen-free liquid then goes down to the shale shaker and back into the pits. The geothermal separator has 8-in. inlet and outlet flow lines, and the inside of the separator is lined to reduce erosion.
Data acquisition equipment on-site recorded flow-out temperature and pressure. Also monitored were nitrogen injection pressure, temperature and flow rate. Nitrogen pump pressure must be high enough to entrain nitrogen in the stand pipe. All data was available and transmitted via the rig-site WITS network.
Float subs were inserted to the top of the drill string to prevent the upward migration of nitrogen when the rig pumps were turned off. This increased safety, reduced wasted nitrogen and reduced time spent bleeding the drill string when making a connection.
Drilling Procedures
A number of drilling procedures were drawn up aimed at increasing the preparedness of the rig crew for events that could occur and aid steps to reach TD as quickly as possible without taking shortcuts. These issues had to be addressed before operations commenced as many personnel were being exposed to closed-loop and hydrostatic balance manipulation methods for the first time. This was a critical step toward ensuring personnel and equipment safety on the rig site and mitigating drilling hazards.
Drilling with nitrified fluid creates scenarios that conventional drilling operators may not be familiar with. Therefore, procedures were translated into German and circulated to the relevant people.
In addition to normal UBD operation procedures, rig crew were presented with the information that would allow them to react to equipment failures, well control and ESD events in which the presence of nitrogen would be a factor to consider. Another critical factor to account for was the communication between rig floor and the nitrogen injection crew. Standard rules for radio communication and reporting were established.
Operations
The GT 1 open-hole section was drilled in two phases using four UBD runs. Initially, UBD Runs 1 and 2 were drilled from the 10 ¾-in. liner shoe at 3,664-meters to 4,503-meters MD. A subsequent acid job and well test proved unsatisfactory, so the UBD separation and nitrogen injection packages were rigged up again. UBD Runs 3 and 4 were drilled from 4,505-meters MD to 4,937-meters MD.
UBD Run 1
This run was conducted from 18-25 February 2011. A 3-meter rat hole was drilled beyond the 10 ¾-in. liner. The run initially started well with full returns and a low
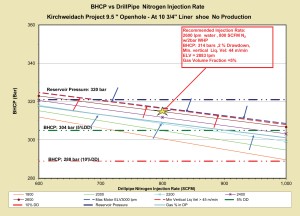
nitrogen rate, which kept the operation slightly overbalanced. Nitrogen injection rates were gradually increased to 14 cu meters/min and held steady at this rate as the UBD system was effective in lowering the equivalent circulating density.
Although this is below the initial model predictions, this left room to increase if desired. On 23 February, it was found that LWD transmissions were very noisy, and signals were not received with a nitrogen flow rate over 12 cu meters/min. A compromise was made to maintain flow rates to ensure adequate data transmission from the tool to the surface. This effectively increased the ECD and ESD, and the system was not truly underbalanced at all times, but it enabled the rig to continue drilling in the given circumstances.
Foaming issues became a problem on 19 February due to the reaction of the drilling fluid polymer (xantin gum) with nitrogen. The initial solution of adding a defoaming agent proved to temporarily solve the issue, but the problem persisted and the system became unmanageable.
The decision was taken to completely replace the drilling fluid in the pits with fresh water without any polymer. Although this was not ideal, returns were recorded on surface, and it helped decrease the daily costs for drilling fluid.
On 25 February, with ROP consistently low at 1 meter/hr, the decision was made to pull out of hole and change the bit. At this point, the bit had spent 96 hrs on bottom. An average instantaneous ROP of 11.6 meters/hr across for this run was recorded, which was decreased by the time spent drilling with the greatly deteriorated bit.
UBD Run 2
A second UBD run was started with a new bottomhole assembly run in hole on 26 February. On this occasion, LWD signal transmission was greatly improved at nitrogen injection rates of 16 cu meters/min. LWD data transmission was lost on 27 February at a depth of 4,219-meters MD. Neotec calculated ECD and bottomhole pressure in line with LWD output prior to end of transmission. The decision was taken to continue to drill ahead without MWD directional guidance.
From here on, knowledge of bottomhole conditions was solely based on the calculated model, which until this point had tracked MWD readings with great satisfaction. For this run, increased emphasis was placed on pit volume tracking. It was in this bottomhole section that significant formation fluid gains were taken while drilling UBD as the reservoir was induced to flow to surface.
Increased torque was experienced while backreaming before connections from a depth of 4,320-meters MD. TD was called at 4,503-meters MD on 2 March 2011. Due to the lack of MWD guidance, the planned hole trajectory was not properly followed. Cave systems and pronounced fractures along the well path explain periods of diverse drilling parameters and pit volume changes.
Traditionally, cave systems add complexity to UBD jobs as they can cause both high fluid gains and losses at surface. These may have been a location of temporary cuttings storage. On flowing the reservoir when pulling out of hole and during the wiper trip, this may have been a source of cutting re-injection back into the annulus.
An average instantaneous ROP of 8.5 meters/hr was recorded for this run.
UBD Run 3
UBD Run 3 started after the stimulating and test work done in the well gave unsatisfactory results, and the decision was made to extend the well to try to reach the main fault. This time CPD was paramount to get returns while circulating as the losses were above 140 cu meters/hr, and the available supply of water was 60 cu meters/hr.
Early attempts to initiate full conventional circulation failed, with the rate of fluid losses to formation too high to maintain the required surface pit volume to continue drilling. Drilling eventually commenced with the sourcing of additional water supply.
On 17 April, annular injection started with a two-phase fluid of water and nitrogen being pumped between the 20-in. surface casing and 13 3/8-in. concentric casing. As this operation progressed, nitrogen injection was gradually increased as drilling fluid injection was decreased. This continued until it was possible to just pump nitrogen in the annular cavity.
The rig pumps were then realigned to start pumping drilling fluid down the drill string, and rotary drilling commenced. This dual-injection method worked initially with optimal rates of 2,000 lpm of drilling fluid and 10 cu meters/min of nitrogen.
A decision was made to investigate the effect of increasing annular nitrogen injection from 10 cu meters/min to 20 cu meters/min. This proved less optimal, and the nitrogen rate was returned to 10 cu meters/min. However, this had the effect of essentially super-charging the annular cavity with nitrogen. As this nitrogen rounded the concentric casing perforations, high-pressure slugging resulted in the well blowing itself dry. Concentric casing injection was halted, and nitrogen was realigned to pump down the drill string.
The presence of this concentric casing, however, was beneficial for the fact that the annular pressure drop was decreased, making it easier to lift cuttings out of the hole.
At 4,540-meters MD, a short trip was performed, and the string was pulled back to 3,555-meters MD. Annular nitrogen injection was halted, and the operation resumed with just drill pipe injection. Injection rates of 2,000 lpm drilling fluid and 10 cu meters/min nitrogen remained optimal values for maintaining adequate fluid return rates to continue drilling. Return rates were typically 50% of volume pumped, which was typically calculated to be a loss of 60 cu meters/hr. The high loss rate is attributed to the acidizing job that was performed after UBD Run 2. The increase in size of fissures and fractures led to increased permeability. A high proportion of fluid pumped from surface was lost to the formation, with nitrogen moving to the high side of the horizontal section, where it too was mostly lost to formation. It is believed some volume of nitrogen did return to surface, but this was very minor with respect to the volume injected. However, the presence of the nitrogen was responsible for decreasing the hydrostatic head sufficiently that some formation fluid influx was induced in the open-hole section above acidized zone.
Further, nitrogen prevented sticking at tight spots along the well path that developed in the later stages of UBD Run 2.
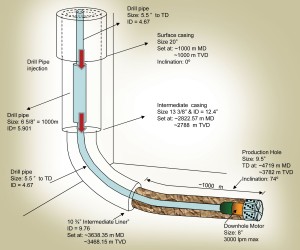
At 4,670-meters MD, drill pipe injection rates were increased to 2,500 lpm and 15 cu meters/min. A bit trip was called at 4,726-meters MD. The average instantaneous ROP for UBD Run 3 was 15.3 meters/hr.
UBD Run 4
UBD Run 4 drilling commenced with fluid and nitrogen injection rates varying from 2,300 lpm to 2,500 lpm and 15 to 20 cu meters/min respectively. This run was rather uneventful compared with UBD Run 3. Average ROP for the section was 9.6 meters/hr. Improved returns were viewed, and this is likely due to the eventual plugging of fractures and fissures, as well as formation of skin on borehole walls. A number of pills were pumped after TD, and this helped clean the hole of a large quantity of cuttings.
The drill string became stuck while pulling out of hole, and the re-introduction of nitrogen was found to aid the recovery. Reduction of differential sticking is a long-recognized benefit of UBD and previous wells in this locality have all run into pipe stick problems at shallower depths.
The temperature of returns at surface was noticeably below that experience on UBD Runs 1 and 2. This is a strong indicator that water was being produced from above the acidized zone at shallower depths. LWD data is the best source for bottomhole temperature comparisons. TD was called at 4,937-meters MD (3,793.3-meters TVD) at 17:35 on 27 April 2011. An additional concern with the rig was the drill string weight approaching the maximum pulling capability of the rig.
Lessons Learned
Problems stemmed from the MWD/LWD failure in UBD Run 2 and the decision to drill on. Several points were noted regarding the tool build, and MWD/LWD tool performance at high pump rates for UBD Runs 3 and 4 was greatly improved. Electromagnetic measurement-while-drilling tools were cost-prohibitive but would have not suffered annular fluid composition-related interference. In the end, improved tool design was sufficient, and perfect detection was recorded at the elevated pump rates. Additionally, the mud motor was changed from 6 5/8 in. for UBD Runs 1 and 2 to 8 in. for UBD Runs 3 and 4. This also enabled a greater motor throughput, raising the ELV.
While concentric casing injection was not a success in this case, its presence in the annulus for UBD Runs 3 and 4 indicated further analysis needs to be done for the concentric drilling method before applying it in the future.
Without accurate flow detection rigged up on the flow line, watching pit volume gains and losses is crucial to understanding the downhole performance of the system.
Foaming was not initially accounted for and provided some adverse drilling conditions. Preemptive and aggressive defoaming is essential for nitrified drilling fluid operations. In a very active system this is not always possible, but it is highly recommend.
Using concentric casing carries a risk, which may not be worth the investment in rig modification as the drill pipe injection method used on GT 1 has proven successful. It is strongly advised to employ this method on future UBD wells in this region.
The application of multiple float subs and NRV’s greatly reduced time spent bleeding nitrogen from the drill string once the rig pumps were shut down. The GT 1 well introduced UBD technology to the rig crew and other service companies, which inevitably caused some confusion and problems, especially when adding the language barrier between the rig crew and the UBD crew. This is expected to greatly improve in future operations where the rig crew has a better understanding of the equipment and techniques used during UBD operations.
The knowledge that the UBD crew has acquired of the rig and the location will also aid in improving future operations.
Conclusion
The scope of this operation was to successfully drill the GT 1 well underbalanced through the Malm reservoir carbonates to a depth of 4,720-meters MD to evaluate and exploit the geothermal properties of the reservoir. After four UBD runs, TD was called at 4,937-meters MD. The expected test results were not achieved on the first attempt, but after extending the well to its final TD of 4,937 meters, the well objectives were accomplished.
The use of CPD was a key factor for the efficient drilling of the extension.
UBD techniques enabled GT 1 to achieve a greater depth than any known well previously drilled in this locality. Additionally, the ability to achieve 100% returns is a vast improvement over conventional techniques previously applied in the area. Total loss situations were avoided on UBD Runs 1 and 2.
Ultimately, UBD permitted GT 1 to be drilled to a point where well testing could be possible with reduced formation damage due to the invasion of drilling fluid solids.
While the original well path was changed, drilling the longest open-hole section in the Malm reservoir allowed it to hit all the planned targets, providing significant information about the target reservoir. Moreover, extending the well TD to 4,937-meters MD in the Malm reservoir allowed a significant achievement by hitting the main fault in the area at +/- 4,900-meters MD. Achieving this objective will greatly aid future drilling in the region as well.
All of this would have not been possible without the aid of nitrified drilling fluid mitigating drilling hazards and lowering the annular hydrostatic pressure head.
The injection of nitrogen into an annular space created with a concentric casing string needs to be carefully planned and considered in the well design, otherwise it will lead to problems with the surface equipment due to irregular underbalanced conditions.
The use of annular pressure and temperature sensors can greatly assist in the determination of the rate of nitrogen to be pumped during drilling and can show influx/loss zones.
Although CPD was not used in the GT 2 and GT 2a wells, it was ready to be deployed and was considered as the technical solution to continue drilling if severe losses would have appeared. It is recommended to include early in the planning stages of the well design in geothermal projects in the area the use of CPD as an option to allow the reaching the well objectives in case total losses appear.
This article is based on SPE/IADC 156895, “Successful Controlled Pressure Drilling Application in a Geothermal Field,” 2012 SPE/IADC Managed Pressure Drilling and Underbalanced Operations Conference and Exhibition, Milan, Italy, 20–21 March 2012.